Challenges and Opportunities for Incorporating Climate Change’s Impacts on Ocean Systems into the Social Cost of Greenhouse Gases
This report summarizes a series of RFF workshops on incorporating ocean impacts into the social cost of greenhouse gases.
Abstract
The social cost of greenhouse gases (SC-GHG) is an estimate of the economic cost to society of an incremental metric ton of emissions of a given greenhouse gas. Recent advances in SC-GHG estimates represent a major step forward towards a comprehensive accounting of the impacts of greenhouse gases, yet they still omit important impacts of climate change such as its effects on ocean ecosystems and fisheries. As a step towards incorporating ocean system impacts into SC-GHG estimates, researchers at Resources for the Future (RFF) and their colleagues convened a group of 40 scientists and policymakers for a series of three strategic workshops. The first workshop served to level-set participants on state-of-the-art SC-GHG modeling, using the RFF-Berkeley Greenhouse Gas Impact Value Estimator (GIVE) model as an example. The second and third workshops focused on two topics identified as priority areas: coral reefs and fisheries. Key topics of discussion included: i) available statistical techniques well suited to the complexities of ocean ecosystems and economies, ii) the importance of the compounding effects of multiple stressors such as temperature increases, extreme events, and ocean acidification on coral reefs and how to capture them in projections of future climate scenarios, and iii) approaches to modeling recreational and commercial fisheries while accounting for economic dynamics such as substitution effects and hedonic adaptation, as well as resource-driven geopolitics. This report documents the dialogue from the workshops, serving as reference material for continuing collaboration to incorporate ocean impacts into more comprehensive SC-GHG estimates.
1. Introduction
Significant progress has been made in recent years on improving the scientific basis for estimates of the economic impacts of climate change (Carleton et al., 2022; NASEM 2017; Rennert, Errickson, et al., 2022; EPA 2023). However, recent state-of-the-art estimates of the social cost of greenhouse gases (SC-GHG) nonetheless still omit many categories of climate change impacts, such as impacts on ocean systems. Closely connected with the atmosphere and acting as a sink for most of the heat trapped by the greenhouse effect, oceans are essential to life for humankind. While the scientific understanding of climate change’s effects on fisheries and other economic and social institutions tied to the oceans is continually improving, the existing research base offers pathways to begin incorporating ocean impacts into SC-GHG estimates with the knowledge we possess today.
To shed light on this subject, we conducted a series of interviews and workshops with experts from a broad range of economic and environmental science disciplines related to the oceans. In the interviews, we sought individual perspectives on where to focus our conversations about economic valuation of ocean impacts, as well as referrals for other researchers to include in the workshops. Altogether, we sat down with over a dozen experts from the US Environmental Protection Agency (EPA), National Oceanic and Atmospheric Administration (NOAA), Scripps Institution of Oceanography, University of British Columbia, University of Oregon, Vrije Universiteit Amsterdam, and several other institutions. Evaluating topics based on both the anticipated magnitude of their associated economic damages and the feasibility of capturing those damages accurately within an SC-GHG estimation framework (typically an integrated assessment model), most experts gravitated towards two focus areas: coral reefs and fisheries. These two topics formed the structure of each of our two workshops, which were preceded by a preliminary session in which we familiarized the invited experts with the SC-GHG methodology and literature. Each workshop was attended by approximately 30 leading researchers and policymakers from around the world, including at least one expert actively working on each topic. Their enthusiastic engagement demonstrates the ubiquitous acknowledgement of this topic’s importance among the broader environmental science and environmental economics communities (see next page).
In this report, we summarize the information conveyed at the workshops in a streamlined format, setting a course for future collaborative work to build SC-GHG damage functions covering climate’s effects on coral reefs and fisheries.
Workshop Participants
- Joshua Abbott, Arizona State University
- Bernardo Bastien-Olvera, Scripps Institution of Oceanography
- Keith Brander, Technical University of Denmark
- Luke Brander, Vrije Universiteit Amsterdam
- Ken Caldeira, Carnegie Institution for Science
- Nicholas Cassar, Duke University
- William Cheung, University of British Columbia
- Stephen Colt, University of Alaska Anchorage
- Sarah Cooley, Ocean Conservancy
- Frank Errickson (co-moderator), Princeton University
- Katharina Fabricius, Australian Institute of Marine Science
- Eli Fenichel, Yale University
- Chris Free, University of California, Santa Barbara
- Steve Gaines, University of California, Santa Barbara
- Corinne Hartin, US EPA
- Cora Kingdon, University of California, Berkeley
- Gunnar Knapp, University of Alaska Anchorage
- Kailin Kroetz, Arizona State University
- Vicky Lam, University of British Columbia
- Lisa Levin, Scripps Institution of Oceanography
- Chris Moore, US EPA
- Daiju Narita, University of Tokyo
- Ernie Niemi, Natural Resource Economics, Inc.
- Michael Oppenheimer, Princeton University
- Stephen Pacella, US EPA
- Jim Palardy, The Pew Charitable Trusts
- Malin Pinsky, University of California, Santa Cruz
- Brian Prest (organizer and moderator), Resources for the Future
- Lisa Rennels, University of California, Berkeley
- Kevin Rennert (co-moderator), Resources for the Future
- Katharine Ricke, Scripps Institution of Oceanography
- Eric Roberts, The Nature Conservancy
- Alex Rogers, REV Ocean
- James Sanchirico, University of California, Davis
- Jeffrey Shrader, Columbia University
- Rashid Sumaila, University of British Columbia
- Travis Tai, Pacific Climate Impacts Consortium
- Richard Tol, University of Sussex
- Donn Viviani, Climate Protection and Restoration Initiative
- Jordan Wingenroth (co-moderator), Resources for the Future
2. Workshop #1: Integrated Assessment Modeling
In the first workshop, which took place on October 27, 2023, Brian Prest (Resources for the Future, RFF) presented an overview of the RFF-Berkeley Greenhouse Gas Impact Value Estimator (GIVE) model, an integrated assessment model (IAM) designed to estimate the SC-GHG. GIVE includes four different categories of climate impacts: temperature-related mortality, agriculture, energy use, and sea level rise (Rennert, Errickson, et al., 2022). These sectors were chosen as focus areas because of earlier research suggesting they likely comprise the most important monetized climate damages.
Here, we will briefly summarize the GIVE model to provide context about how ocean impacts could be incorporated in the SC-GHG. We will also describe the discussion from this workshop along with the research cited therein, both of which set the stage for the specific coral-reef and fisheries subtopics on which the subsequent workshops focused.
2.1. The GIVE Model
GIVE is built on the collaborative work of researchers from many different scientific disciplines, combining projections of socioeconomic variables and greenhouse gas emissions, climate models, climate impact estimates, and methods of discounting future impacts to present values. This approach yields SC-GHG estimates grounded in empirical research and communicable assumptions (Figure 1). A major design aspect of the GIVE model is its use of Monte Carlo analysis via random sampling to allow for SC-GHG values to reflect uncertainty as described in the supporting climate science and economics literature.
Figure 1. The framework of the GIVE IAM
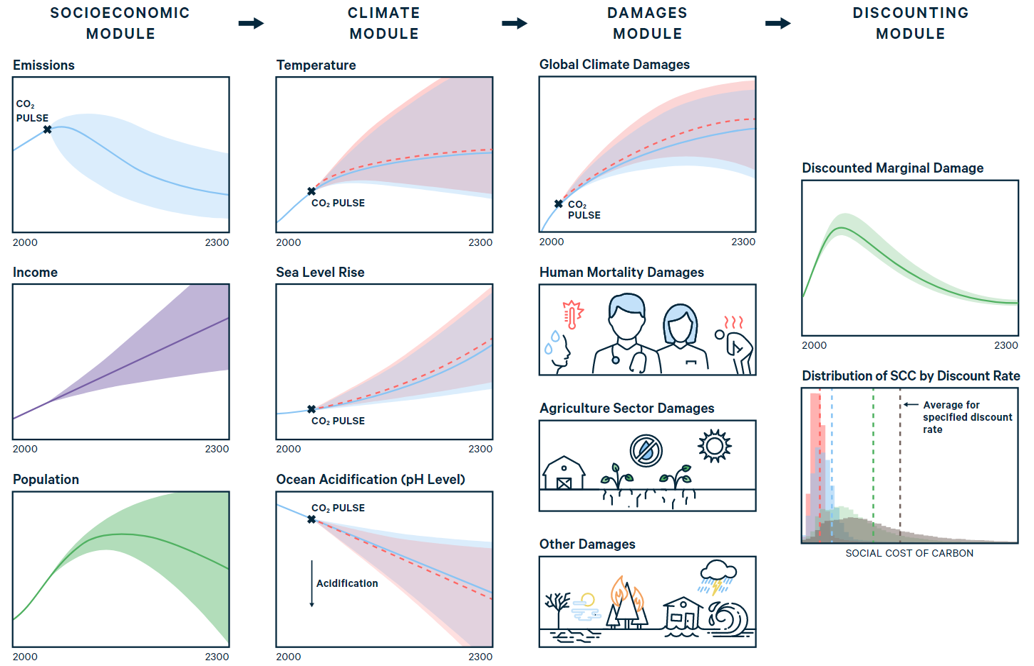
The RFF Socioeconomic Projections (RFF-SPs) used in GIVE, represented in the first column of Figure 1, include forecasted population (Raftery & Ševčíková, 2023), economic growth (Müller et al., 2022), and greenhouse gas emissions (Rennert, Prest, et al., 2022), extending to year 2300 as is necessary to capture the bulk of discounted climate damages values with an appropriate discount rate (Newell et al., 2022). Population and economic growth are projected at the country level to account for regional variation in the magnitude of climate impacts, factors which are sure to be similarly relevant for assessing climate change’s impacts on fisheries and coral reefs.
Greenhouse gas emissions from the RFF-SPs feed into the Finite Amplitude Impulse Response (FaIR) model, a simple, parsimonious climate model designed to emulate global temperature responses (second column of Figure 1) to greenhouse gas emissions from more computationally intensive physical simulations (Smith et al., 2018). The FaIR model represents the carbon cycle, where carbon dioxide emissions are offset to some degree by land and ocean carbon sinks. The FaIR model improves on earlier models, such as those used in the IPCC Fifth Assessment Report, by calibrating the behavior of carbon sinks to depend on cumulative carbon uptake and concurrent temperatures. It also models the oxidation of methane into carbon dioxide, and other chemical processes. It was one of several emulators included in the IPCC Sixth Assessment Report (IPCC, 2023), and GIVE uses the version of FaIR (v1.6.2) calibrated for that report.
Temperature and ocean heat content, two output variables from FaIR, subsequently feed into a sea-level-rise model called BRICK (Building blocks for Relevant Ice and Climate Knowledge, represented in the second column of Figure 1), which accounts for ice melt from Greenland, Antarctica, and elsewhere, and which also downscales global sea level projections to regional values (Wong et al., 2017). BRICK is currently used by GIVE to estimate direct effects of sea level rise on coastal communities, but it may also be well suited for estimating other ocean impacts such as coral reef loss.
Currently, GIVE estimates climate impacts associated with agriculture, heat-related mortality, the energy sector, and sea level rise (third column of Figure 1). Each of these damage sectors involves a damage function, which takes socioeconomic and climate variables as inputs and returns estimates of economic impacts in dollars (Figure 2). The socioeconomic variables in GIVE are typically tied to the RFF-SPs. For example, heat-related mortality depends on national birth and death rates, which are taken from the RFF-SPs as population projections. Damage functions also depend on climate variables. Current GIVE damage sectors only depend on temperature and sea level rise, but new damage functions tied to oceans may involve other outcomes, such as ocean heat content or acidity.
After estimating damages, the final two steps for calculating the SC-GHG are to estimate marginal damages and discount them to present day values (fourth column of Figure 1). Marginal damages are those associated with a small pulse of additional emissions in a single year, on top of historical and projected total emissions. This is the appropriate method for calculating the SC-GHG so that it can be used for benefit-cost analysis in decisions about emissions reductions or increases that are small relative to total global emissions. Lastly, damages are discounted to present-day value and aggregated. GIVE uses a Ramsey-like discounting method—where discount rates are tied to future economic growth—as was recommended by the National Academies of Science, Engineering, and Medicine (2017).
Figure 2. The generic structure of a damage function
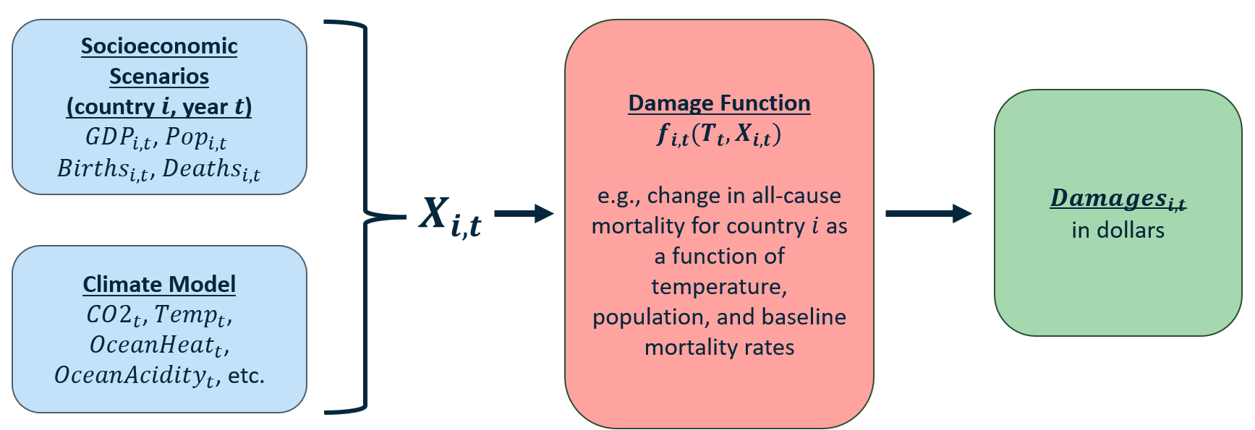
2.2. Group Discussion
After the presentation on the GIVE model and the typical structure of damage functions, attendees participated in an open discussion focused on the pathway towards building one or several damage functions to incorporate ocean systems into SC-GHG calculations. Specific focus areas included assessing the difficulties that may arise when seeking to place economic value on oceanic impacts of climate change, identifying studies on ocean systems with desired methodological characteristics of damage functions (Diaz & Moore, 2017), and comparing market and nonmarket valuation approaches for sectors such as fisheries and coral-reef tourism.
The conversation began with a focus on fisheries. Relative to the broad agroeconomic literature, which includes many studies focused on crop yields and related economic effects of climate change (e.g., Bindi & Olesen, 2011; Hatfield et al., 2011; F. C. Moore et al., 2017), studies on fisheries do not often directly relate temperature (or other climate outcomes) to economic effects in quantitative terms. For example, there is a fair amount of literature on how climate change is shifting the regions inhabited by fish (e.g., Bell et al., 2013; Pinsky et al., 2020), but several workshop participants emphasized that the ecological mechanisms by which climate change affects fisheries are far more difficult to understand than effects of temperature and precipitation on terrestrial agriculture. One attributed this to the practical difficulty of studying relatively inaccessible oceanic habitats and the complexity of the ecosystems on which many commercial fish and shellfish species depend. As evidence of this lack of understanding, they pointed to the historic decline in Alaskan snow crab following the 2018–2019 marine heat wave in the Bering Sea. Although greater understanding of the mechanisms involved is now emerging (e.g., Szuwalski et al., 2023), the decline largely surprised the scientific community, fishermen, and policymakers alike. This led to the first ever closure of the Alaskan snow crab season in 2022, which was recently extended for a second year with dim prospects for reopening in the near future.
Whereas other damage sectors may be captured accurately with reduced-form models, it is possible that fisheries may be better suited to an integrated modeling approach. This involves linking several models together, each representing individual sub-processes involved in the broader relationship(s) covered by the model as a whole. This type of model may help to address path dependency, which is the way in which welfare impacts at a given temperature in the future depend on socioeconomic and climate trajectories that led to it. Successful region-specific examples of integrated models such as the Alaska Climate Integrated Modeling (ACLIM) Project demonstrate the strengths of this approach (NOAA 2023b). However, the path forward for building such a model on the global scale, which naturally entails a greater degree of complexity, is yet to be determined.
Other challenges associated with developing a fisheries damage function include the difficulty of estimating producer welfare/surplus, the role of adaptation strategies such as aquaculture, and the paucity of global data on fisheries. Studies have been carried out estimating effects of climate change on specific fisheries in specific geographic regions (e.g., C. Moore et al., 2021; Narita & Rehdanz, 2017). However, it is not entirely clear how such research could be accurately scaled up to the breadth required for a global IAM in terms of taxonomy, geographic range, drivers of ecosystem change, and economic roles affected (including producers).
As for coral reefs, discussion began with a somewhat technical question about the best spatial resolution for damage functions. The GIVE model estimates sea-level-rise damages using the Coastal Impact and Adaptation Model (CIAM), which estimates effects for 12,000 individually parameterized coastal segments (Diaz, 2016). For more far-reaching and less accessible deep-sea ecosystems, which includes many of the world’s largest fisheries, and for damages based on nonuse value, modeling communities with a high degree of geographic precision is not especially important. However, for damages that affect local communities specifically, such as lost tourism revenue due to coral bleaching, accurately calculating monetary damages requires taking economic attributes of nearby communities into account. A workshop participant working on geographically specific damage functions covering coral reefs suggested that it may be possible to implement such an approach on top of the infrastructure present in the CIAM model, but the methodology for doing so requires a good deal more thought.
Many important modeling questions—and anticipated challenges—apply to developing damage functions for both coral reefs and fisheries, along with other ocean-related impacts. One example that was discussed during the first workshop is ocean acidification, which affects many of the same ecosystems and economies as temperature change. Ocean pH and ocean temperature are difficult to decouple because of their collinearity. Both are tied to increases in atmospheric carbon dioxide concentrations. However, ocean acidification has an outsized impact on marine invertebrates, including both corals and commercially exploited shellfish, because it reduces their ability to amass calcium carbonate in their structures and exoskeletons (Feely et al., 2004). These effects translate into considerable economic costs (Narita & Rehdanz, 2017). Because chemical effects of temperature and ocean acidity on these organisms are mechanistically related (Tai et al., 2021), multi-stressor models composed of structural, biological equations are the industry standard. However, these models raise complicated statistical questions tied to multicollinearity and path dependence, which require careful consideration when developing techniques to create reduced-form emulators suitable for use within an IAM.
Along with global drivers related to climate change, such as ocean acidification and temperature increase, regional climate impacts such as arctic sea ice loss also have implications for a wide range of economic sectors and stakeholders (Alvarez et al., 2020; O’Garra, 2017). Fisheries and shipping routes currently blocked by sea ice may become more accessible, which may in some cases result in economic benefits. However, these same effects could contribute to geopolitical conflicts with negative effects on society (Brutschin & Schubert, 2016), which may be especially difficult to value in SC-GHG estimates. Moreover, physical effects of melting arctic sea ice and Greenland glaciers may affect the Atlantic Meridional Overturning Circulation (Sévellec et al., 2017), which drives ocean currents around the world and which may have a direct effect on fisheries (Nye et al., 2011) and coral reefs (Elliot et al., 2019). While feedback cycles and interactions between some climate-related phenomena have been represented in IAMs (e.g., Dietz et al., 2021), there is much room for improvement in terms of comprehensiveness and in terms of the accuracy of process-based models for ocean-related ecological and economic systems.
While these challenges might seem intimidating, discussion at the first workshop also explored some promising paths towards accounting for ocean impacts in IAMs. For instance, on the ocean acidification question, the collinearity between ocean temperature and pH may be less of a problem for reduced-form damage functions and over long time horizons if their joint effect is the driver of interest. However, addressing the impacts of the two variables as a whole, without differentiating between the two, precludes the use of process-based multi-stressor models as described above. Doing so would also overlook differences between the two variables in terms of their short-term and region-specific fluctuations. However, the statistical challenges caused by the collinearity of the two variables go away when they are not decoupled. For the long timescales involved in IAMs such as GIVE, a simple parameterization based on the combined effects of the two factors may yield a reasonably accurate SC-GHG estimate.
Nonparametric models, or “surfaces,” also overcome some of the challenges described above. Advances in computational power since the earliest iterations of models estimating economic effects of climate change (e.g., Nordhaus, 1991) allow for complex structural models to be run over a wide range of future socioeconomic, emissions, greenhouse gas concentration, and temperature trajectories with a suitable resolution and within a reasonable timeframe. Statistical interpolation techniques can then be used to fill out the multidimensional parameter space of these input variables. In a Monte Carlo simulation for a broader IAM, damages at different coordinates along the time, greenhouse gas concentration, temperature, and economic axes can be estimated not with an algebraic equation but rather with more of a lookup function. This is an intriguing area for future research and may present a solution to some of the problems posed by path dependence, tipping points, bifurcations, and other nonlinearities commonly arising in conversations about the complex and highly uncertain future of Earth’s oceans.
3. Workshop #2: Coral Reefs
For the second workshop, held on November 17, 2023, Drs. Ken Caldeira and Luke Brander opened the discussion with presentations on the impacts of climate change on coral reefs and the ecosystem services that they provide. Dr. Caldeira’s presentation focused on the impacts of increasing marine temperatures and ocean acidification on coral reef habitats, and how the paleo-record can inform our understanding of coral reef health under different climate futures. Dr. Brander next focused on how climate change’s impact on the ecosystem services provided by coral reefs—fisheries, coastal protection, recreation, and nonuse value to name a few—can be monetized and incorporated into SC-GHG estimates. Based on the two presentations and the informative group discussion that followed, this section discusses the science of coral reefs under a changing climate, economic approaches to valuing climate impacts on coral reefs, and the challenges behind developing a global coral reef damage function.
3.1. Climate Change and Coral Reefs
Warming waters and ocean acidification pose a grave threat to the world’s coral reef ecosystems. To date, oceans have absorbed roughly 90 percent of the excess heat in the climate system, with global sea-surface temperatures warming by roughly 0.88°C (IPCC, 2023). This increasing thermal stress from warmer surface waters can lead to mass coral bleaching events, where corals eject the symbiotic algae that give them their bright colors. The corals are then left with a “bleached” white appearance and substantially increased susceptibility to disease and overall mortality risk. In addition, the ocean’s uptake of carbon dioxide from the atmosphere is causing seawater to become more acidic. This makes it difficult for corals to maintain or build up their calcium carbonate skeletons. Alongside the effects of warming waters and ocean acidification, corals are also vulnerable to nonclimate threats such as coastal pollution and sediment deposits due to land use practices (Cramer et al., 2020; NOAA 2023a), hence these background factors should be considered when modeling the impact of climate change on coral reef.
The marine geologic record suggests that coral reefs could adapt to hotter waters if the rate of warming occurs slowly enough. Tens of millions of years ago, coral reefs still extended along the equator when surface water temperatures were much warmer than today (Jones et al., 2022). Yet while changes in the deep past occurred at rates measured in millions of years, today’s rate of warming can be measured in terms of decades, which is potentially much too fast for many corals to adapt (Brown et al., 2023). However, this does not necessarily mean that coral reefs will disappear immediately. While repeated coral bleaching events have led to coral mortality and algae overgrowth in many areas, there has also been a resurgence of coral cover in some areas in recent years; particularly in the northern Great Barrier Reef (AIMS, 2022). Recent work has also shown that remote coral reef systems in the Pacific Ocean have been developing an improved thermal tolerance of approximately 0.1°C per decade (Lachs et al., 2023). The overall trend for corals will likely exhibit considerable geographic variation, with substantial differences between coral reef extent and coral reef diversity (Pandolfi et al., 2011).
However, the marine geologic record also shows that reef ecosystems have never persisted across time in waters with the same chemical composition as is projected to occur under some of the most plausible future CO2 emission pathways (Cao & Caldeira, 2008). In general, as ocean acidification increases, there will be less calcium carbonate available for corals to utilize when building up their skeleton structures. Scientists commonly use the aragonite saturation state of sea water as a coral-relevant measure of ocean acidification, as aragonite is one of the most abundant, soluble forms of calcium carbonate found in seawater. Corals tend to do well and reproduce when aragonite saturation states are above 3, but become stressed at lower values and can even begin to dissolve when saturation states fall below 1 (NOAA 2015). For atmospheric CO2 concentrations as low as 450 ppm, research suggests a majority of the world’s coral reef ecosystems will face declining aragonite saturation states. At 550 ppm, a majority of the world’s corals could become stressed (saturation states below 3) and virtually no coral reef will be surrounded by waters with saturation states comparable to the pre-industrial ocean (Figure 3). Overall, coral reef damage functions will need to capture both the near-term stress from warming waters as well as the longer-term threat ocean acidification poses to reef ecosystem stability and extent.
The complexity of coral vulnerability, including geographic variation and the potential for thermal adaptation through evolutionary changes, calls into question how best to model coral and climate impacts for SC-GHG estimates. Current damage functions often estimate future climate impacts in terms of an aggregate metric such as annual global average surface temperature (Rennert, Errickson, et al., 2022). However, coral reef mortality is closely associated with a more granular metric known as degree heating weeks, which measures a reef’s accumulated thermal stress above a particular temperature threshold for a given length of time. For instance, NOAA’s Coral Reef Watch provides daily degree heating week estimates for the world’s coral reefs that capture “instantaneous bleaching heat stress…during the most recent 12-week period” (NOAA n.d.). Coral reefs also exhibit considerable path dependence in their vulnerability. Three bleaching events in quick succession will have a very different effect on coral stress and mortality as compared to three separate bleaching events spread evenly throughout the year. Given the importance of reef location, evolution, and the timing of extreme warming events, an alternative damage function approach could embed a process-based model of coral reefs within an IAM (Lane et al., 2013). The process-based model would then account for the key biological processes of coral reefs at appropriate temporal and spatial scales, with the damages subsequently being monetized and aggregated into the broader IAM framework. While this approach offers promise, global process-based models of coral mortality and adaptation at the reef level are not yet available.
Aragonite saturation state and coral reefs (from Cao & Caldeira, 2008)
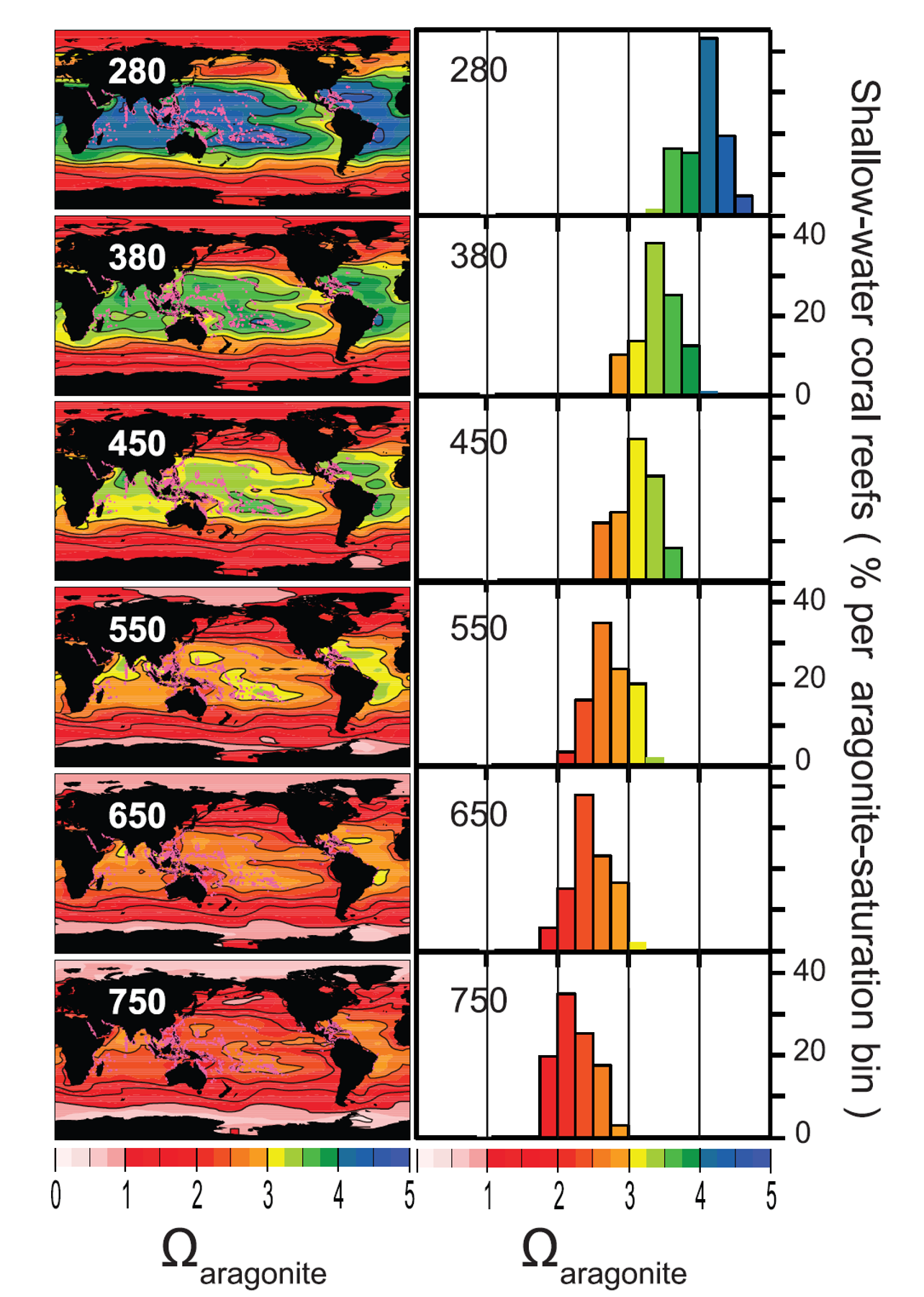
Note: (left) Maps of model-predicted aragonite saturation states at different atmospheric CO2 stabilization concentrations (ppm), plotted alongside existing shallow-water coral reef locations (shown as magenta dots). (right) Percentage distribution of modern-day coral reefs at each aragonite saturation bin under different atmospheric CO2 stabilization concentrations. Aragonite saturation value at each reef location is interpolated from nearby open ocean values simulated by the model. Results are obtained by adding model-predicted perturbations to modern observations, except for the Arctic Ocean where results are derived directly from model simulations due to the lack of observations.
Reprinted from Cao & Caldeira 2008, © 2008 American Geophysical Union
3.2. Coral Reefs and Ecosystem Service Valuation
Assigning a dollar value to the impact climate change has on coral reef ecosystems and the services they provide requires modeling a complex chain of effects, starting with a release of CO2 emissions and ending with a change in human welfare brought about by climate’s impacts on the reefs themselves. This impact pathway can be broken up into four distinct modeling components that an IAM would have to capture to properly account for and value coral reef damages:
- The multiple stresses facing coral reefs: As discussed above, coral reefs face a variety of climate and nonclimate stressors that a damage function would have to account for. In addition to warming surface waters and ocean acidification, other stressors include over-fishing, pollution runoff, sea level rise, land use change, and invasive species.
- Changes in coral reef characteristics and conditions: Estimating the impact of climate change on coral reefs requires quantifying the condition of coral reefs at highly local scales, their sensitivity to climate change and other stressors, and how these reefs may be affected and/or adapt over time. Specific relevant reef characteristics that an IAM should capture include reef extent and coverage, biodiversity levels, the health and condition of the reef, and reef rugosity (a measure of surface roughness that can also serve as an indicator of overall biodiversity levels).
- The ecosystem services provided by coral reefs over time: Coral reefs provide a number of ecosystem services that benefit society. Examples of services include supporting fisheries and as a nutrition source, protecting coasts from erosion and storm damage, providing tourism and recreational/educational opportunities, and nonuse values (values people assign to the existence of reef ecosystems even if they do not directly or indirectly benefit from the reef itself). As climate change affects coral reefs over time, the ecosystem services they provide will likely be impacted as well.
- Economic valuation of coral reef ecosystem services: To align with the other sectoral damage functions that inform bottom-up SC-GHG estimates, a coral reef damage function will have to assign a monetized value to the benefits that reef ecosystems provide. This value should be expressed in terms of the total change in human welfare that is attributable to changes in multiple ecosystem services combined.
Attempts to value coral reef ecosystem services face two major hurdles. First, the value of coral reef ecosystem services exhibits considerable spatial variation that also depends on the local supply and demand for these services. As discussed above, no model to date can capture local reef conditions and ecosystem services for the entire globe. Second, many coral reef ecosystem services are nonmarket goods. Because these services are not traded directly through markets, they lack a corresponding price that could directly inform estimates of the welfare derived from them. Instead, researchers must rely on imperfect nonmarket valuation methods that can include surveying people about how much they would be willing to pay for a particular coral reef service (stated preference methods), collecting data on how much people are willing spend to visit a reef or to live in proximity of one (revealed preference methods), and using data on the cost of damages to infrastructure in the absence of reefs (avoided damage cost method) or cost of replacing services provided by reefs (replacement cost method). These nonmarket valuation methods are subject to a number of limitations and potential biases. A meta-analysis of 166 published coral reef valuation studies (Brander et al., 2007) found that the type of nonmarket valuation method used can have a significant effect on the estimated recreation benefits of coral reefs, with the commonly used contingent valuation approach providing among the lowest recreational benefit estimates (Figure 4). As this example highlights, coral reef damage functions and their associated uncertainty levels concerning future impacts will be highly sensitive to the underlying valuation methods they are informed by.
The question of how to carry out long-term environmental valuations when society’s future preferences may change poses another challenge for developing a coral reef damage function. Future generations may simply place a different value (higher or lower) on the disappearance of a coral reef compared to the value for reefs that society holds today. The inherent uncertainty associated with how societal preferences may change poses a nontrivial challenge for researchers. One potential solution looks at how income or scarcity effects impacted the value of reef ecosystem services in the past (generally, increasing incomes or reductions in the supply of ecosystem services both serve to increase the monetized value of reefs on the margin). However, this approach yields highly uncertain estimates because it is challenging to accurately predict future changes in human preferences based on past preference changes alone.
An additional concern relates to the concept of hedonic adaptation, whereby the novelty of some change (both positive or negative) wears off over time until an individual’s preferences adapt to that original change. There has been a wide array of research on hedonic adaptation for nonenvironmental changes, highlighting the impermanent effects of major events such as winning the lottery or facing a personal health crisis (Kahneman et al., 1999). In the coral reef context, future generations may simply become used to a state of the world in which coral reefs are substantially degraded or disappearing, the same way today’s society has to some degree become inured to the disappearance of the dodo bird or the loss of the Dutch elm. While perhaps contentious, this element of human psychology and the change in society’s preferences over time could significantly influence the valuation of changes to coral reefs and the ecosystem services they provide.
Figure 4. Coral reef recreation values by region, recreational activity, and valuation method (from Brander et al., 2007)
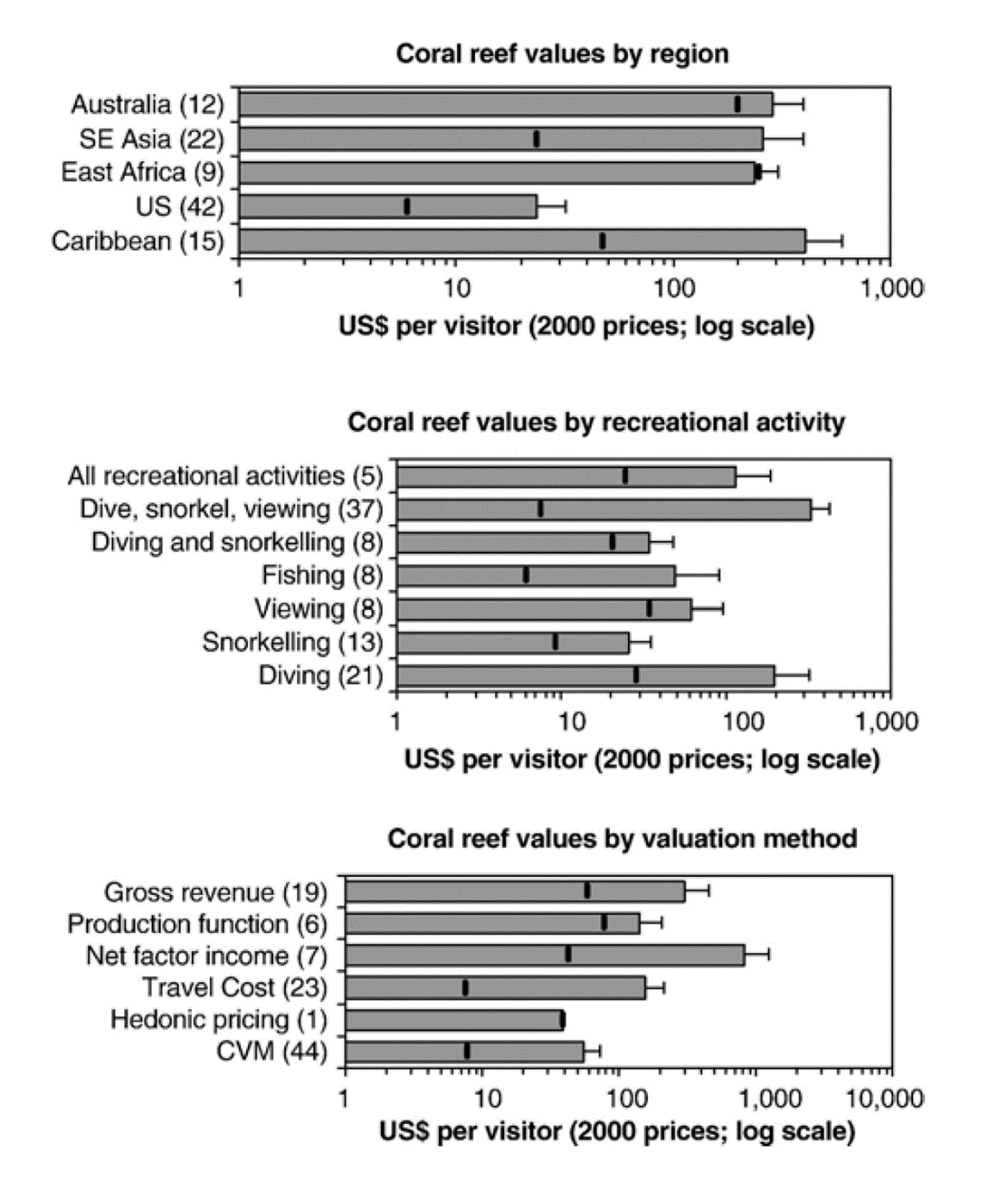
Note: The bars represent the mean value, the dots represent the median value and the error bars represent the standard error of the mean. The numbers in brackets are the number of observations for each category.
Reprinted from Brander et al. 2007, © 2007 Elsevier B.V.
The issues discussed above highlight a number of research priorities associated with developing a global coral reef damage function. These include:
- The effect of extreme events: Coral reefs are vulnerable to extreme events such as marine heat waves and hurricanes. Modeling how these extreme events impact coral reefs and how they will evolve under various alternative pathways of future climate change represents a key component of projecting future harms to coral reef ecosystems.
- Accounting for uncertainty of coral reef damages: Each stage of the chained modeling framework central to a coral reef damage function (coral stressors, coral conditions, changes in ecosystem services, and economic valuation) contains a host of uncertainties that will propagate throughout an IAM. A careful accounting of these various uncertainties and their interactions, particularly the effect of different nonmarket valuation methods, represents a high priority.
- Modeling individual coral reef ecosystem services: Standard damage functions may only look at a simplified statistical relationship between global average surface temperature and coral reef ecosystem services. However, increases in computing power, satellite data, and the number of coral valuation studies may allow for the development of separate damage functions for individual coral reef ecosystem services. This would represent a more sophisticated, process-based modeling approach that can provide more detail about which coral reef impact channels have the largest effect on SC-GHG estimates.
- Capturing society’s changing preferences for ecosystem services: To date, little research exists on how the value society places on coral reefs may vary into the future due to concepts like hedonic adaptation. Applying this concept to ecosystem services, and coral reefs in particular, represents a promising avenue for future research.
- Equity and coral reefs: Communities along many of the world’s coastlines rely on coral reefs for food and income security. The loss of coral reefs could result in a large decline in the well-being and resilience of these communities, even if the monetized losses may be small. Future research should further explore the link between climate change, coral reefs, and inequality.
While these topics represent interesting avenues for longer-term research, key near-term needs for developing an initial coral reef damage function includes ensuring any such function (i) has global coverage, (ii) is spatially and temporally resolved enough to capture the key channels through which climate change impacts coral reefs, and (iii) accounts for the key uncertainties that would most influence damage estimates. Until coral reef damages are incorporated into IAMs, they will effectively—and incorrectly—be assigned an SC-GHG value of $0.
4. Workshop #3: Fisheries
At the final workshop, held on December 1, 2023, Drs. Chris Moore, William Cheung, and Rashid Sumaila gave presentations on the scientific literature related to impacts of climate change on fisheries. Dr. Moore’s presentation centered on two aims: broadly assessing recent scientific literature on this topic and identifying the types of studies that are still needed to fill gaps as we work towards incorporating ocean fisheries impacts into SC-GHG calculations. Subsequently, Dr. Cheung and Dr. Sumaila presented their model as an example of how to quantitatively predict some of the damages that should be captured in such an SC-GHG estimate. Here, we outline the main points of both presentations, also summarizing the productive, open-ended group discussion that gave other workshop attendees the opportunity to weigh in.
4.1. Literature Review
In general, SC-GHG estimates should aim for a global scope and a comprehensive appraisal of different avenues by which climate affects social outcomes, with impacts expressed in terms of monetized changes to social welfare. By highlighting the respective strengths and limitations of a number of different approaches, Dr. Moore opened the conversation about leveraging the existing literature to develop such a global, comprehensive, and equitable damage function.
Fisheries research occurs over a broad range of spatial scales, with both global (e.g., Cheung et al., 2021; Free et al., 2019; Sumaila, Ebrahim, et al., 2019; Tai et al., 2021) and regional (e.g., Fernandes et al., 2017; Le Bris et al., 2018; C. Moore et al., 2021; Morley et al., 2018) studies presenting findings relevant for developing an SC-GHG model. Generally, regional granularity is an ideal characteristic for IAM components because it allows national and subnational demographic and economic projections to be matched more precisely with the local environmental and ecological data of greatest relevance (Diaz & Moore, 2017). Often, meta-analyses are used to combine many local studies into regional models, which themselves are more directly integrated into IAMs.
Modeling fisheries requires accounting for interregional dynamics due to species migration and range shifts. This makes it more akin to modeling the impacts of agriculture, which entail shifts in the location and magnitudes of crop yields and their consequences for trade flows (e.g., F. C. Moore et al., 2017), as contrasted to situations where interregional interactions are less important—for example, when evaluating climate change’s effects on human mortality (e.g., Cromar et al., 2022). Importantly, a recent comparison of several different leading fisheries models demonstrates that there is still work to be done both in understanding these dynamics (Tittensor et al., 2021) and in creating predictive models with the global consistency and regional specificity that are both required for making robust SC-GHG estimates.
In addition to considering geographic extent, it is important to ensure that damage estimates for SC-GHG models are comprehensive in other dimensions. For instance, studies estimating climate effects on commercial harvests vary in terms of which species and physicochemical drivers they account for. Some use large databases covering hundreds of species that constitute the vast majority of commercial fish harvests (e.g., Lam et al., 2016; Morley et al., 2018), whereas others may cover fewer species (e.g., C. Moore et al., 2021; Weatherdon et al., 2016) or focus on specific subtaxa (e.g., Narita & Rehdanz, 2017; Tai et al., 2021). Most studies covering many different species are constrained to a particular geographic region (e.g., Morley et al., 2018), and while others may have global scope (e.g., Lam et al., 2016), their analyses generally depend on reconstructed datasets that interpolate gaps in fish harvest data using ecological models and information on political agreements and demographics (e.g., Pauly & Zeller, 2015). Workshop participants were generally in agreement that consistent “boots on the ground” annual catch data were generally only available for certain large Western countries. In terms of drivers, studies may cover warming (e.g., C. Moore et al., 2021; Morley et al., 2018), acidification (e.g., Mangi et al., 2018; Narita & Rehdanz, 2017), or both (e.g., Cheung et al., 2021).
Impacts of climate change on recreational fisheries are substantial (Dundas & von Haefen, 2020), but literature on the subject is more limited than for commercial fisheries. Dr. Moore shared that in informal conversations with his colleagues, some have expressed informed opinions that the monetized value of climate change’s impacts on recreational fisheries could be on the same order of magnitude as those on commercial fisheries. Further, in wealthy countries there is good data on willingness to pay for recreational fishing trips at the species level, suggesting a promising area of research for monetizing impacts on species migration. However, broader and more rigorous stated preference surveys and revealed preference studies are required to substantiate those hypotheses.
In addition to the measures of comprehensiveness described above, studies vary in terms of their modeling approaches for both the ocean ecosystems on which fisheries depend and the economies to which they contribute. For modeling oceanographic and ecological aspects, popular approaches include structural equation models, which account explicitly for biological processes (e.g., Cheung et al., 2021), and reduced-form statistical models that relate abundance of fish to climate variables and other hypothesized drivers by applying regression analysis to historical data (e.g., Morley et al., 2018). Both types of models can output population estimates for fish, which inform estimates of catch in economic models used to value fisheries in the context of the broader economy.
Some additional climate phenomena are predicted to affect ocean ecosystems but are not often captured by work based on either of these modeling approaches. Ideally, future research should be conducted to understand how the possible collapse of the Atlantic Meridional Overturning Circulation (AMOC) would affect ecological dynamics and food webs, effects which would also be expected to propagate into economic models and SC-GHG estimates.
Models about the economics of fisheries vary in terms of how impacts are valued, as well as whether projections of economic variables into the future account for predicted changes in real income and the possibility of substitution of other protein sources for fish. Generally, impacts affecting producers are measured in terms of lost revenue (e.g., Fernandes et al., 2017), although some studies also consider losses in terms of employment (e.g., Cheung et al., 2021). Because an SC-GHG estimate is intended to reflect costs inflicted on society as a whole, economic models that estimate effects on consumer welfare are most applicable, suggesting that studies that focus on revenue may be insufficient for measuring welfare impacts. To see why revenue effects are inappropriate measures of human welfare, consider a hypothetical climate-induced loss in fishery supply. This loss clearly reduces human welfare as fewer fish are available for sale and consumption, but in theory it could nonetheless increase fishers’ revenues if fish prices increase more in percentage terms than supply decreases. Thus, using metrics of consumer and producer surplus when estimating damages would be a more appropriate approach (e.g., Narita & Rehdanz, 2017).
When considering United States fisheries specifically, the estimated present value of social welfare losses associated with thermal habitat change can add up to billions of dollars (C. Moore et al., 2021). Ocean acidification, not included in those estimates, may be responsible for approximately $1 billion more in damages via its effects on shellfish production in Europe specifically (Narita & Rehdanz, 2017). Expanding these regional estimates using global models is an important step towards estimating an SC-GHG for fisheries, because losses to one region from habitat migration is offset in at least part by benefits in the destination region. A considerable portion of global damages likely occur along the coasts of Asia, South America, the Caribbean, and Oceania, regions whose inclusion will be imperative for a complete and comprehensive SC-GHG. However, access to good fisheries data is often poor in those regions, making comprehensive estimates challenging to produce.
4.2. An Example of a Global Model
After Dr. Moore described the broader context of fisheries modelling, Dr. Cheung and Dr. Sumaila went into greater depth about the model they developed to predict global ecological and economic effects of climate change on fisheries. To model climate effects on fish populations, they used a dynamic bioclimate envelope model (DBEM). This type of model predicts the effects of climate change on fishery dynamics (e.g., fish body size, mortality, migration) while accounting for habitat characteristics such as temperature, dissolved oxygen levels, acidity, salinity, and sea ice extent, along with physiological and life-history characteristics of fish species, and the abundance of invertebrate and phytoplankton nutrition sources (Cheung et al., 2008, 2011). These factors are condensed into geospatial predictions of inhabitance and abundance for each fish species, which are ultimately used to generate projections of future maximum catch potential (MCP, in units of metric tonnes) and species turnover for various global warming trajectories.
Results from DBEMs suggest that high-emissions scenarios result in decreased MCP and increased species turnover relative to low-emissions scenarios, reflecting reduced fish stocks and shifts in fish habitat (Cheung et al., 2016). Net global decline is in line with ecological theory, which would suggest that declines in net primary production and phytoplankton biomass due to climate change in more heavily fished areas (Bell et al., 2013; Sarmiento et al., 2004) put a ceiling on the energy available for consumption by higher trophic levels, including commercial fish species (Chassot et al., 2010). It is important to acknowledge that while the literature is broadly in agreement about the detrimental effects of the highest-temperature trajectories, such as RCP 8.5 (Gattuso et al., 2015; Lam et al., 2020), some research suggests that more moderate temperature increases might increase MCP in certain tropical areas due to changes in fish migration patterns (Bell et al., 2013), as well as in regions close to the poles due to increased primary production (Barange et al., 2014).
In their model, fish biomass and MCP are used as inputs for a supply-demand model (Sumaila, Tai, et al., 2019). All factors except for price and MCP are held constant, and an inverse relationship is assumed between the two variables (i.e., decreasing MCP results in increasing price). The model accounts for the ways in which the price and demand of different fish affect one another, and it treats income as an additional independent variable. The model treats countries as independent of one another, effectively assuming zero international trade, but its authors assert that their findings would hold with or without that factor and provide evidence from the literature suggesting that the relationship between international and domestic fish prices is weak (Ivanic et al., 2012). On the other hand, that study focused on developing countries and may not capture relationships between developed ones.
Lastly, multiplier approaches (which convert changes in one outcome like revenue to another like income, expenditures, or employment by multiplying by a regionally specific constant) are used to assess effects on seafood workers’ income and household seafood expenditures based on the outputs of the supply-demand model. These account for the linkages between fisheries and the many other facets of a country’s economy. For instance, along the supply chain from a boat unloading at a landing to an entrée at a restaurant or a can on a grocery store’s shelf, fish are transported and sold in bulk, processed in canneries or other facilities, and distributed to restaurants or retailers. Each step involves additional income not captured in records of fishers’ sales quantities and prices using multipliers based on an Input-Output analysis (see Leontief, 1986).
Ultimately, the model concludes that limiting global surface temperature to 1.5°C relative to preindustrial levels—as opposed to a 3.5°C baseline—is expected to increase fishers’ revenue, seafood workers’ income, and savings in household seafood expenditures across all continents and for both developed and developing countries. A few individual countries are predicted to have effects that run counter to the global average, including Russia, Greenland, South Africa, Iran, Pakistan, and others. However, they are the exception to the rule, and the effects of limiting temperature rise are found to be beneficial for fishers, seafood workers, and consumers in most cases (Figure 5).
Figure 5. Projected benefit of meeting the 1.5˚C Paris Agreement target relative to a 3.5˚C baseline (from Sumaila, Tai, et al., 2019)
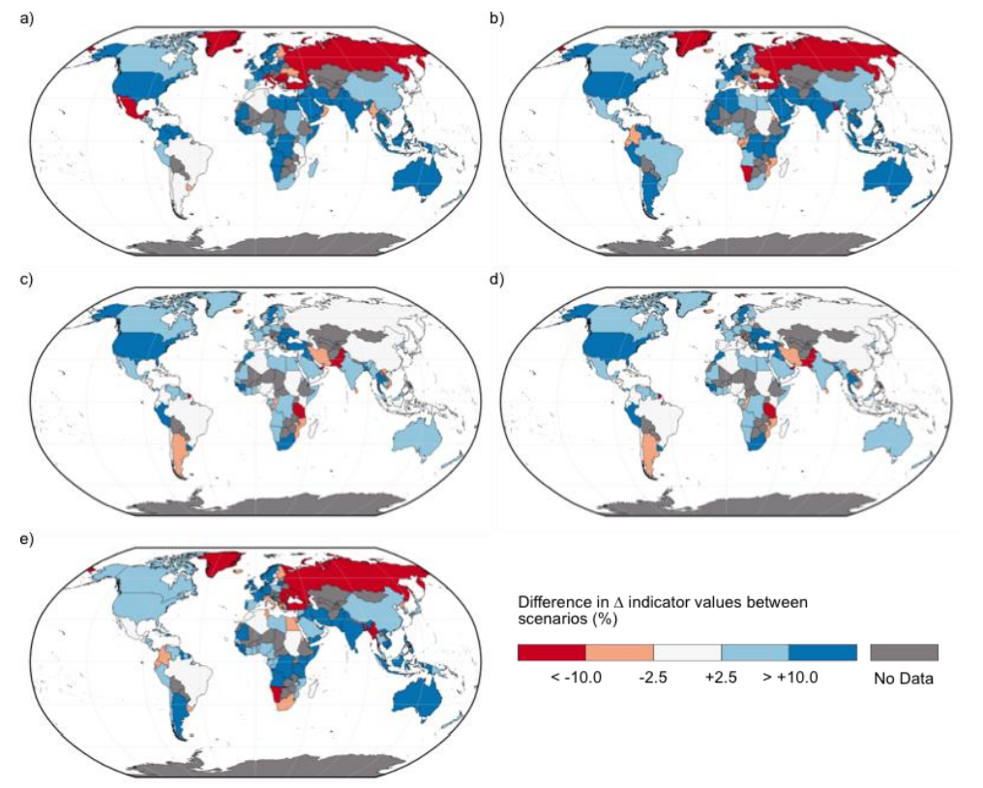
Note: (a) fish biomass, (b) maximum catch potential, (c) fishers’ revenue, (d) seafood workers’ income, and (e) savings in household seafood expenditures
Reprinted from Sumaila, Tai, et al. 2019, © 2019 The Authors, under CC BY-NC license: https://creativecommons.org/licenses/by-nc/4.0/.
Subsequent work has investigated the effect of more severe annual temperature extremes in addition to the multidecadal average temperature increases covered above (Cheung et al., 2021). This demonstrates the versatility of the model and may be an important qualification for its use in IAMs where temperature and other parameters are treated as uncertain variables. However, because most IAMs operate at a yearly resolution, some degree of abstraction may be required to model interannual temperature extremes. Results show that leaving out this aspect may underestimate climate change’s effects on fisheries for many different regions (Figure 6).
Figure 6. Projected changes in (a) the intensity of marine extreme high-temperature events and average sea-surface temperature, (b) their impacts on stock biomass, and (c) their impact on maximum catch potential (from Cheung et al., 2021)
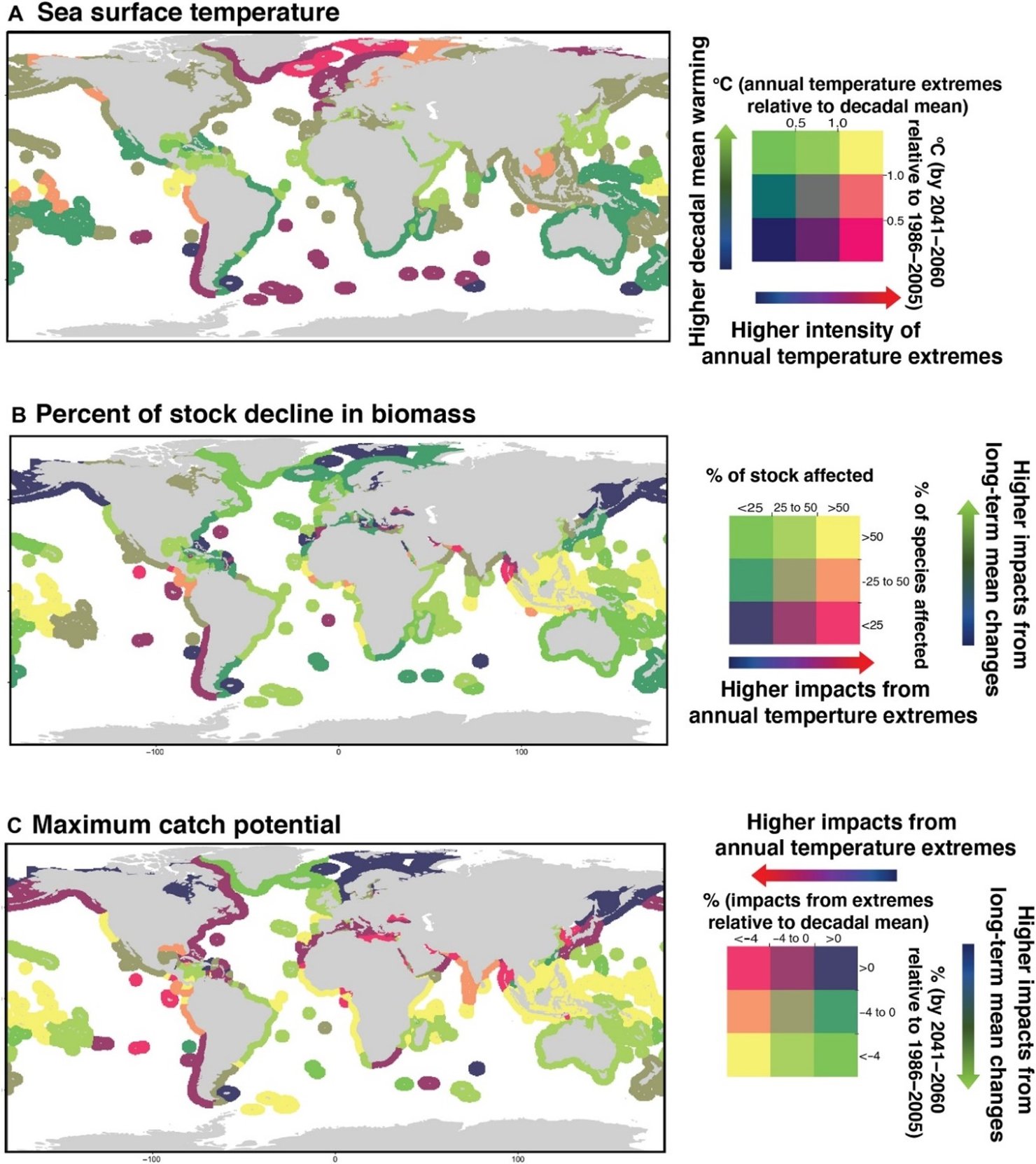
Reprinted from Cheung et al. 2021, © 2021 The Authors, under CC BY-NC license: https://creativecommons.org/licenses/by-nc/4.0.
4.3. Group Discussion
After the presentations, the group dialogued with a focus on developing a fisheries-related damage function for integration into an SC-GHG model. They pulled from their wide-ranging areas of expertise, including fisheries management, statistical modeling of fish stocks, aquaculture, and valuation of climate effects in monetary terms. Limiting factors such as data scarcity in certain regions and types of fisheries were acknowledged, but topics of discussion also acknowledged helpful tools such as existing fishery and ecosystem model platforms. Developing comprehensive global damage functions covering the wide range of possible future climate trajectories is clearly a heavy lift, but this gathering of experts made some progress towards charting a path forward.
Currently, fisheries management is far from ideal in many regions around the world. Climate-induced regional shifts in species abundance and trade flows will also be mediated by future fisheries management policies, which vary around the world. Managed fisheries are likely to be more resilient to climate change than open-access ones. The challenges facing fisheries management in the present day suggest that assuming universal adoption of optimal management decisions in the future within an IAM would likely introduce an optimistic bias in estimates of climate impacts. However, economic models for a wide range of management scenarios are available (Costello et al., 2020). If these were integrated with models of ocean ecology, such as the Fisheries and Marine Ecosystem Model Intercomparison Project, or Fish-MIP (Tittensor et al., 2021), a reduced-form fisheries model could be produced using the statistical techniques described in the section on Workshop #1. One challenge yet to be solved is how to model feedbacks between fisheries management and ocean ecosystem dynamics across the wide range of socioeconomic and climate scenarios involved in making a robust SC-GHG accounting for uncertainty. As with coral reefs, using a simplified model that fails to take nuances such as this into account will likely yield a more accurate SC-GHG estimate than the value of zero implied by using no model at all, as has been the effective valuation used in SC-GHG estimates to date.
A growing source of fish, bivalve, and crustacean food products is aquaculture, the cultivation of such organisms in marine and inland environments. Aquaculture overtook capture fisheries in 2022 as the predominant overall source of aquatic animals for consumption, with almost 90 percent of the growth since 2020 occurring in Asia (FAO, 2024). The aquaculture industry is expected to continue its rapid rate of growth, which could have significant implications for global trade of both fish and land-based agricultural goods (Anderson et al., 2017). It is possible that this expanding food source may help fill nutritional gaps in developing countries left by negative effects of climate change on capture fisheries, although fish cultivation is subject to climate-related losses as well (Cheung et al., 2023). Translating these climate effects on fishery- and aquaculture-based nutrition sources into health-related metrics is a promising avenue for valuation within the context of an SC-GHG model.
Recreational fisheries are also expected to contribute substantially to the total economic value of oceans for the purpose of calculating SC-GHG estimates, but there were several perspectives among participants in the workshop about the relative importance of recreational, commercial, and subsistence fisheries. On the one hand, demand for recreational fishing largely comes from developed countries, whose larger economies in terms of GDP can translate into larger absolute financial losses from climate change for a given marginal change in greenhouse gas emissions—the standard basis for calculating an SC-GHG estimate. However, substitution between recreational fishing sites and/or across alternative types of recreation could offset some of the losses. Substitution also plays an important role in the model of commercial fisheries described above (Sumaila, Tai, et al., 2019) and is an important consideration in subsistence fisheries as well, although alternative options may be limited in some cases. There is also the concept of “hedonic adaptation” to consider, which refers to the notion that people’s expectations change along with their external circumstances. Unlike subsistence and commercial fisheries, where nutrition makes up much of the value of goods, valuation of recreational fisheries is largely based on subjective experiences. A workshop participant presented the allegory that if climate change changed a trout stream into a bass stream, a recreational fisherman born after the fact could be assumed to value fishing bass no less than his predecessor valued fishing trout.
5. Conclusions
With such a wide range of climate mechanisms, ecosystems, and economies involved, comprehensively accounting for ocean-related climate impacts in the SC-GHG presents clear challenges. To address research needs, we assembled a group of experts with a diverse set of research backgrounds, some of whom are already engaged in work on the subject. We discussed several approaches to transforming complex process-based models into reduced forms suitable for use in integrated assessment models. Some experts took inspiration from the model structures used for other economic sectors, such as the agriculture damage function in GIVE. Discussion also covered promising techniques less commonly used in the SC-GHG literature, such as training nonparametric models (or “emulators”) on process-based fishery and coral health models across a wide range of possible future climate and socioeconomic trajectories. Ultimately, different systems will require different approaches. Whereas there already exists a global process-based model for climate effects on fish communities called Fish-MIP (Tittensor et al., 2021), there has yet to be such a model developed for coral reefs, which may be required before some ideas discussed at the workshop can be realized. It was a pleasure spending time with all who attended the workshop, and we hope that this report can serve as a stepping stone towards the collective goal of an accurate SC-GHG estimate for oceanic effects of climate change.